By Contributing Editor Nuala F. Caomhánach
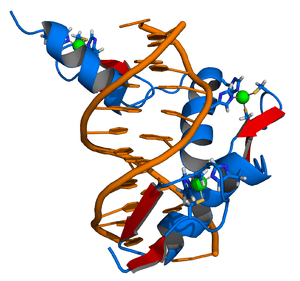
Zinc Finger DNA Complex, image by Thomas Splettstoesser
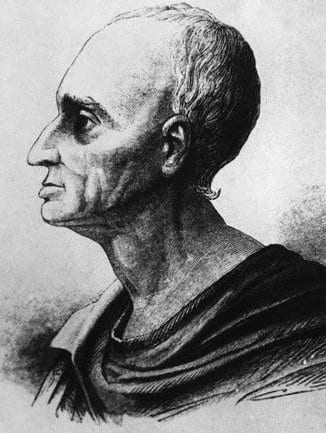
Andreas Sigismund Marggraf
The tale about gag knuckles, taz-two, hairpin, ribbons, and treble clef is quite elusive. Although they sound more like nicknames of a 1920’s bootlegging gang (at least to me) they are the formal nomenclature of a biochemical classification system, known commonly as zinc fingers (ZnF). The taxonomy of zinc fingers describes the morphological motif that the element creates when interacting with various molecules. Macromolecules, such as proteins and DNA, have developed numerous ways to bind to other molecules and zinc fingers are one such molecular scaffold. Zinc, an essential element for biological cell proliferation and differentiation, was first isolated in 1746 by the German chemist Andreas Marggraf (1709-–1782). Zinc fingers were important in the development of genome editing, and while CRISPR remains king, zinc is making a comeback.
Strings of amino acids fold and pleat into complex secondary and tertiary structures (for an overview, see this video from Khan Academy).
[youtube https://www.youtube.com/watch?v=MODnIkQvyz0&w=560&h=315]
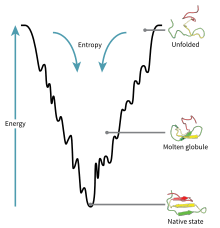
Fig. 1: The folding funnel
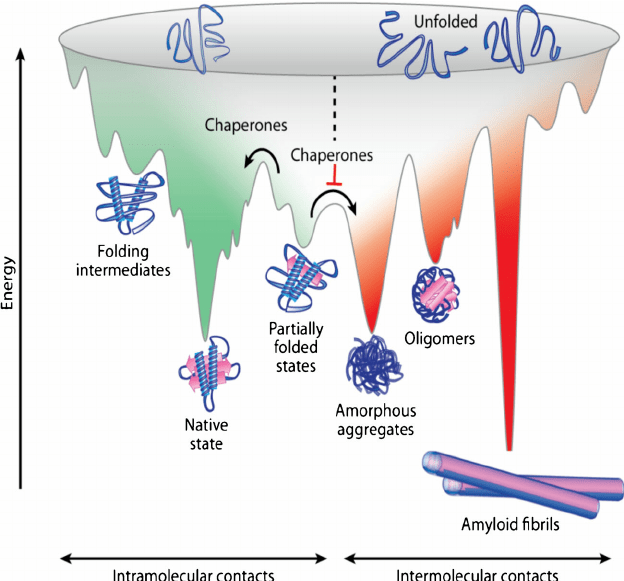
Fig. 2: The energy landscape
Proteins with zinc finger domains—meganucleases—act as molecular DNA scissors, always ready to snip and organize genetic material. The return of these biochemical bootleggers, an older generation of genome editing tools, is due to the problem of exploring the invisible molecular world of the cell. In this age of genomic editing, biologists are debating the concept of protein stability and trying to elucidate the mechanism of protein dynamics within complex signalling pathways. Structural biologists imagine this process through two intermingled metaphors, the folding funnel (fig. 1) and the energy landscape (fig. 2). The energy landscape theory is a statistical description of a protein’s potential surface, and the folding funnel is a theoretical construct, a visual aid for scientists. These two metaphors get scientists out of Levinthal’s Paradox, which argues that finding the native or stable 3-dimensional folded state of a protein, that is, the point at which it becomes biologically functional, by a random search among all possible configurations, can take anywhere from years to decades. Proteins can fold in seconds or less, therefore, biologists assert that it cannot be random. Patterns surely may be discovered. Proteins, however, no longer seem to follow a unique or prescribed folding pathway, but move in different positions, in cellular time and space, in an energy landscape resembling a funnel of irregular shape and size. Capturing the choreography of these activities is the crusade of many types of scientists, from biochemists to molecular biologists.
Within this deluge of scientific terminology is the zinc atom of this story. With an atomic number of 30, Zinc wanders in and out of the cell with two valence electrons (Zn2+). If Zinc could speak it might tell us that it wishes to dispose, trade, and vehemently rid-itself of these electrons for its own atomic stability. It leads with these electrons, recalling a zombie with outstretched arms. It is attracted to molecules and other elements as it moves around the cytoplasm and equally repelled upon being cornered by others. Whilst not as reactionary as the free radical Hydrogen Peroxide (H2O2), it certainly trawls its valency net in the hope of catching a break to atomic stasis, at least temporarily. In this world of unseen molecular movements a ritual occurs as Zinc finds the right partner to anchor itself to. Zinc trades the two electrons to form bonds making bridges, links, ties and connections which slowly reconfigure long strings of amino or nucleic acids into megamolecules with specific functions. All of this occurs beneath the phospholipid bilayer of the cell, unseen and unheard by biologists.
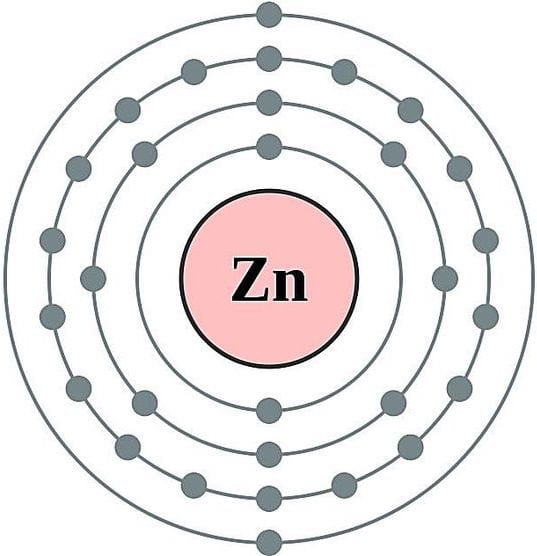
Model of a Zinc atom
The cell itself is an actor in this performance by behaving like a state security system, as it monitors Zn2+ closely. If the concentration gets too high, it will quarantine the element in cordoned-off areas called zincosomes. By arranging chains of amino acids, such as cysteine and histidine, close to each other, the zinc ion is drawn into a protein trap, and held in place to create a solid, stable structure. They connect to each other via a hydrogen atom, with hydrogen’s single electron being heavily pulled on by Carbon as if curtailing a wild animal on a leash. In the 1990s, when the crystal structures of zinc finger complexes were solved, they revealed the canonical tapestry of interactions. It was notable that unlike many other proteins that bind to DNA through the 2-fold symmetry of the double helix, zinc fingers are connected linearly to link sequences of varying lengths, creating the fingers of its namesake. Elaborate architectural forms are created.
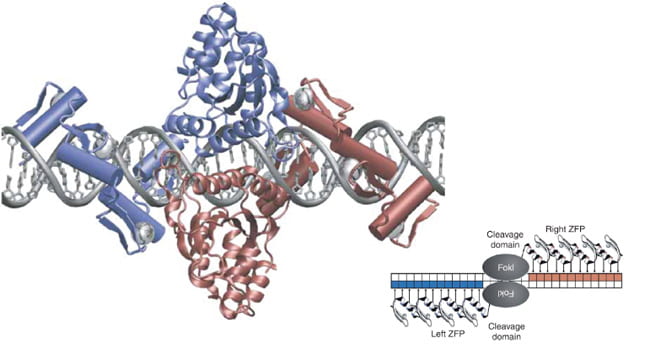
Zinc Finger architecture, image from Miller, J.C. et.al., An improved zinc-finger nuclease architecture for highly specific genome editing. Nature Biotechnology 25, 778-785 (2007).
Like a dance, one needs hairpins, or rather beta-hairpins, two strands of amino acids that form a long slender loop, just like a hairpin. To do the gag knuckle one needs two short beta-strands then a turn (the knuckle), followed by a short helix or loop. Want to be daring, do the retroviral gag knuckle. Add one-turn alpha-helix followed by the beta hairpin. The tref clef finger looks like a musical treble clef if you squint really hard. First, assemble around a zinc ion, then do a knuckle turn, loop, beta-hairpin and top it off with an alpha-helix. The less-complex zinc ribbon is more for beginners: two knuckle turns, throw in a hairpin and a couple of zinc ions. Never witnessed, biologists interpret this dance using x-ray crystallography, data that looks like redacted government documents, and computer simulated images.
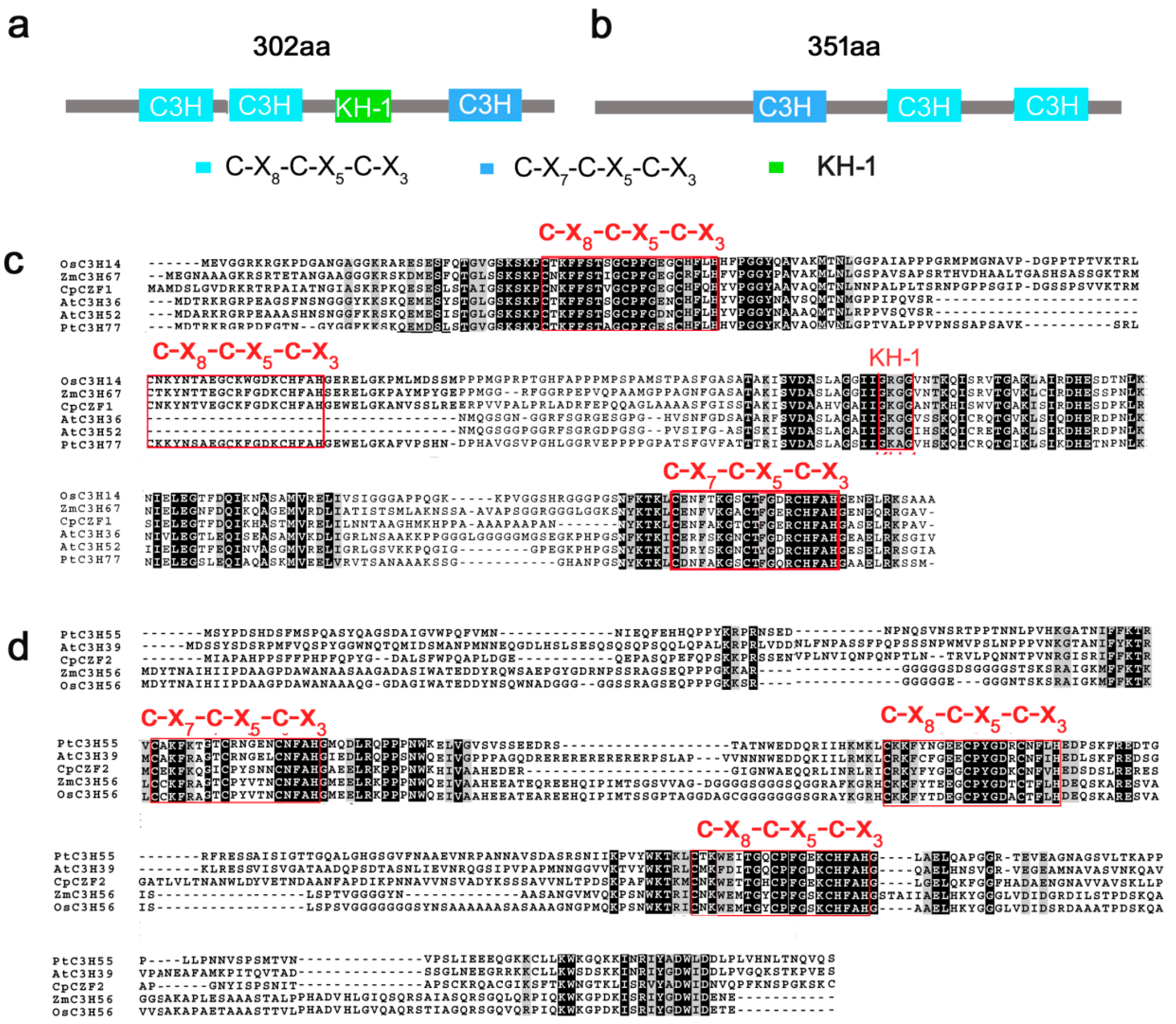
X-ray crystallography of a Zinc Finger Protein, image from Liu et al., “Two C3H Type Zinc Finger Protein Genes, CpCZF1 and CpCZF2, from Chimonanthus praecox Affect Stamen Development in Arabidopsis,” Genes 8 (2017).

Aaron Klug
In the 1980s, zinc fingers were first described in a study of transcription of an RNA sequence by the biochemist Aaron Klug. Since then a number of types have been delimited, each with a unique three-dimensional architecture. Klug used a model of the molecular world that required stasis in structure.The pathway towards this universal static theoretical framework of protein functional landscapes was tortuous. In 1904, Christian Bohr (1855–1911), Karl Albert Hasselbalch (1874-1962), and August Krogh (1874-1949) carried out a simple experiment. A blood sample of known partial oxygen pressure was saturated with oxygen to determine the amount of oxygen uptake. The biologists added the same amount of CO2 and the measurement repeated under the same partial oxygen pressure. They described how one molecule (CO2) interferes with the binding affinity of another molecule (O2) to the blood proteins, haemoglobin. The “Bohr effect” described the curious and busy interactions of how molecules bind to proteins.
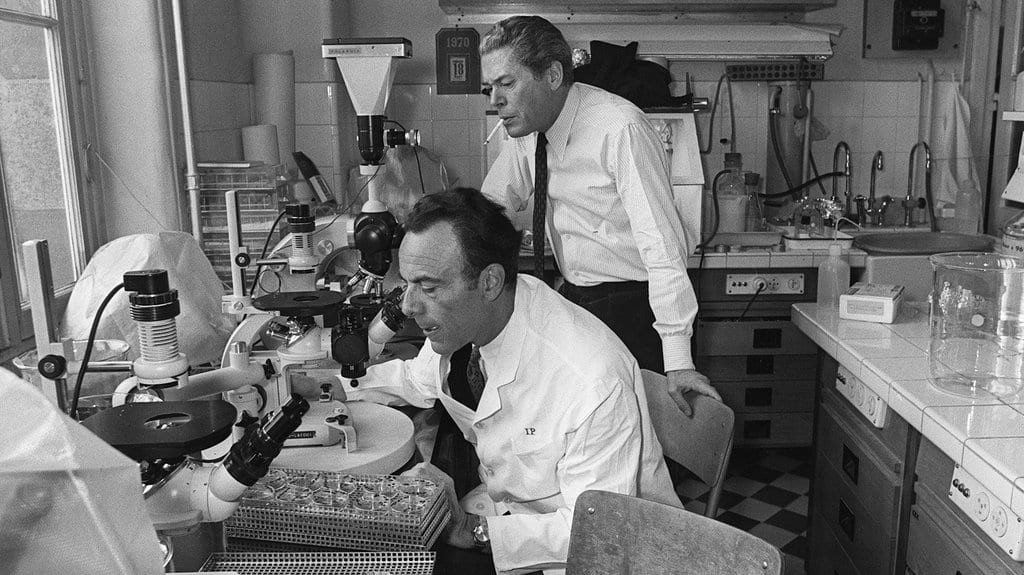
Jacques Monod and François Jacob, image by Agence France-Presse
In 1961, Jacques Monod and Francois Jacob extended Bohr’s research. The word ‘allosteric’ was introduced by Monod in the conclusion of the Cold Spring Harbor Symposium of 1961. It distinguished simultaneously the differences in the structure of molecules and the consequent necessary existence of different sites across the molecule for substrates and allosteric regulators. Monod introduced the “induced-fit” model (proposed earlier by Daniel Koshland in 1958). This model states that the attachment of a particular substrate to an enzyme causes a change in the shape of the enzyme so as to enhance or inhibit its activity. Suddenly, allostery erupted into a chaotic landscape of multiple meanings that affected contemporary understanding of the choreography of zinc as an architectural maker. Zinc returned as the bootlegger of the cellular underworld.
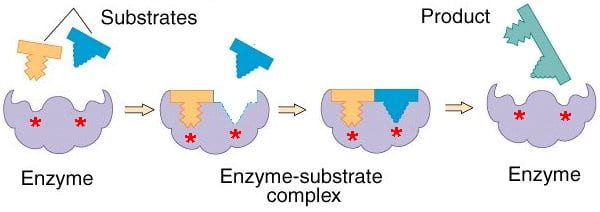
The “induced-fit” model
Around 2000, many biologists were discussing new views on proteins, how they fold, allosteric regulation and catalysis. One major shift in the debate was the view that the evolutionary history of proteins could explain their characteristics. This was brought about by the new imaginings of the space around the molecules as a funnel in an energy landscape. When James and Tawfik (2003) argued that proteins, from antibodies to enzymes, seem to be functionally promiscuous, they pulled organismal natural selection and sexual selection theory into the cellular world. They argued that promiscuity is of considerable physiological importance. Bonding of atoms is more temporal and more susceptible to change than thought of before. Whilst they recognized that the mechanism behind promiscuity and the relationship between specific and promiscuous activities were unknown they opened the door to a level of fluidity earlier models could not contain. Zinc fingers, thus, played an important role in being a “freer” elements with the ability to change its “mind”.
These ideas were not new. Linus Pauling proposed a similar hypothesis, eventually discarded as incorrect, to explain the extraordinary capacity for certain proteins (antibodies) to bind to any chemical molecule. This new wave of thinking meant that perhaps extinct proteins were more promiscuous than extant ones, or there was a spectrum of promiscuity, with selection progressively sifting proteins for more and more specificity. In 2007, Dean and Thornton, reconstructed ancestral proteins in vitro and supported this hypothesis. If the term promiscuity as it has been placed on these macromolecules sticks, what exactly does it mean? If promiscuity is defined as indiscriminate mingling with a number of partners on a casual basis, is zinc an enabler? A true bootlegger? This type of language has piqued the interest of biologists who see the importance of this term in evolution and the potential doors it opens in biotechnology today. Promiscuity makes molecular biology sexy.
To state that a protein, or any molecule, is not rigid or puritanical in nature but behaves dynamically is not the equivalent of stating that the origin of its catalytic potential and functional properties has to be looked for in its intrinsic dynamics, and that the latter emerged from the evolutionary history of these macromolecules. The union of structural studies of proteins and evolutionary studies means biologists have not only (re)discovered their dynamics but also highlights the way that these properties have emerged in evolution. Today, evolutionary biologists consider that natural selection sieves the result, not necessarily the ways by which the result was reached: there are many different ways of increasing fitness and adaptation. In the case of protein folding with zinc fingers, what is sieved is a rapid, and efficient folding choreography. These new debates suggest that what has been selected was not a unique pathway but an ensemble of different pathways, a reticulating network of single-events, with a different order of bond formation.
If the complex signalling pathways inside of a cell begins with a single interaction, zinc plays a star role. Zinc, along with other elements such as Iron, are the underbelly of the molecular world. Until captured and tied down, the new view of proteins offers to bond mechanistic and evolutionary interpretations into a novel field. This novelty is a crucial nuance to explain the functions and architecture of molecular biology. As the comeback king, zinc fingers are used in a myriad of ways. As a lookout, biologists infected cells with with DNA engineered to produce zinc fingers only in the presence of specific molecules. Zinc reports back as biologist looks for the presence of a molecule, such as a toxin, by looking for the surface zinc fingers rather than the toxin itself. Zinc fingers are obstructionists. In 1994 an artificially-constructed three-finger protein blocked the expression of an oncogene in a mouse cell line. As informants, they capture specific cells. Scientists have experimented by creating a mixture of labeled and unlabeled cells and incubated them with magnetic beads covered in target DNA strands. The cells expressing zinc fingers were still attached to the beads. They introduce outsiders into the cell, such as delivering foreign DNA into cells in viral research. In tracking these bootleggers, scientists have pushed the limits of their own theories and molecular landscapes, but have landed on promiscuity, a culturally laden, inherently gendered word.
Leave a Reply